Anilines, which are aromatic compounds containing an amino group, have found their way into numerous drug candidates due to their structural versatility and potential pharmacological benefits. However, their inclusion is not without its limitations and challenges.
Anilines have been utilized in drug discovery for their ability to interact with biological targets and modulate physiological processes. An understanding of why replacing anilines in drug candidates may be necessary requires an exploration of the specific reasons behind their potential drawbacks.
Why replace anilines?
When considering the replacement of anilines in hit compounds, lead or drug candidates, several compelling reasons come to light. One of the primary concerns is metabolic instability. Anilines are often susceptible to various metabolic enzymes in the body, which can lead to rapid excretion of the drug, reducing its effectiveness. Moreover, anilines have been associated with toxicity issues and the potential for adverse side effects.
While anilines are found in the chemical structures of several on the market drugs, it's important to note that the presence of an aniline group does not necessarily imply toxicity or adverse effects. Anilines are just one component of these complex molecules, and the overall safety and efficacy of these drugs depend on their complete chemical structures and how they interact with the body. In some cases, chemical modifications may be explored to improve the properties of these drugs or to reduce any potential issues associated with the aniline moiety. Additionally, modifying anilines can lead to increased receptor selectivity, therefore reducing off-target effects (and its related toxicity).
In this article, we describe a research workflow for exploring alternatives to anilines and their replacement with other chemical groups. We demonstrate how Cresset Discovery’s team can apply their deep knowledge and computational chemistry techniques such as Cresset's bioisosteric replacement tool Spark™,1 to optimize the efficacy and safety profiles of drug-like small molecules.
How to replace anilines?
The ChEMBL Database was interrogated to find the most suitable drug(s) to be used as starting points for this study. From this search, 187 FDA-approved drugs were found containing at least one aniline in their chemical structures. These were further analyzed and only 19 representatives of discrete chemical series (by cluster analysis) were identified. Among these, Bromfenac was selected due to the presence of aniline in the central ring of the core, whereas Amprenavir bears an aniline moiety as an end-point substituent. Moreover, while Bromfenac is a small molecule, Amprenavir shows a PK profile with Molecular Weight in the high range and slightly poor solubility. Bromfenac was used as a reference for a ligand-centric experiment, since no available crystal structure with COX-2 has been published, while Amprenavir has been co-crystalized with its pharmacological target, HIV Protease, and will be used as a reference for a structure-based analysis.
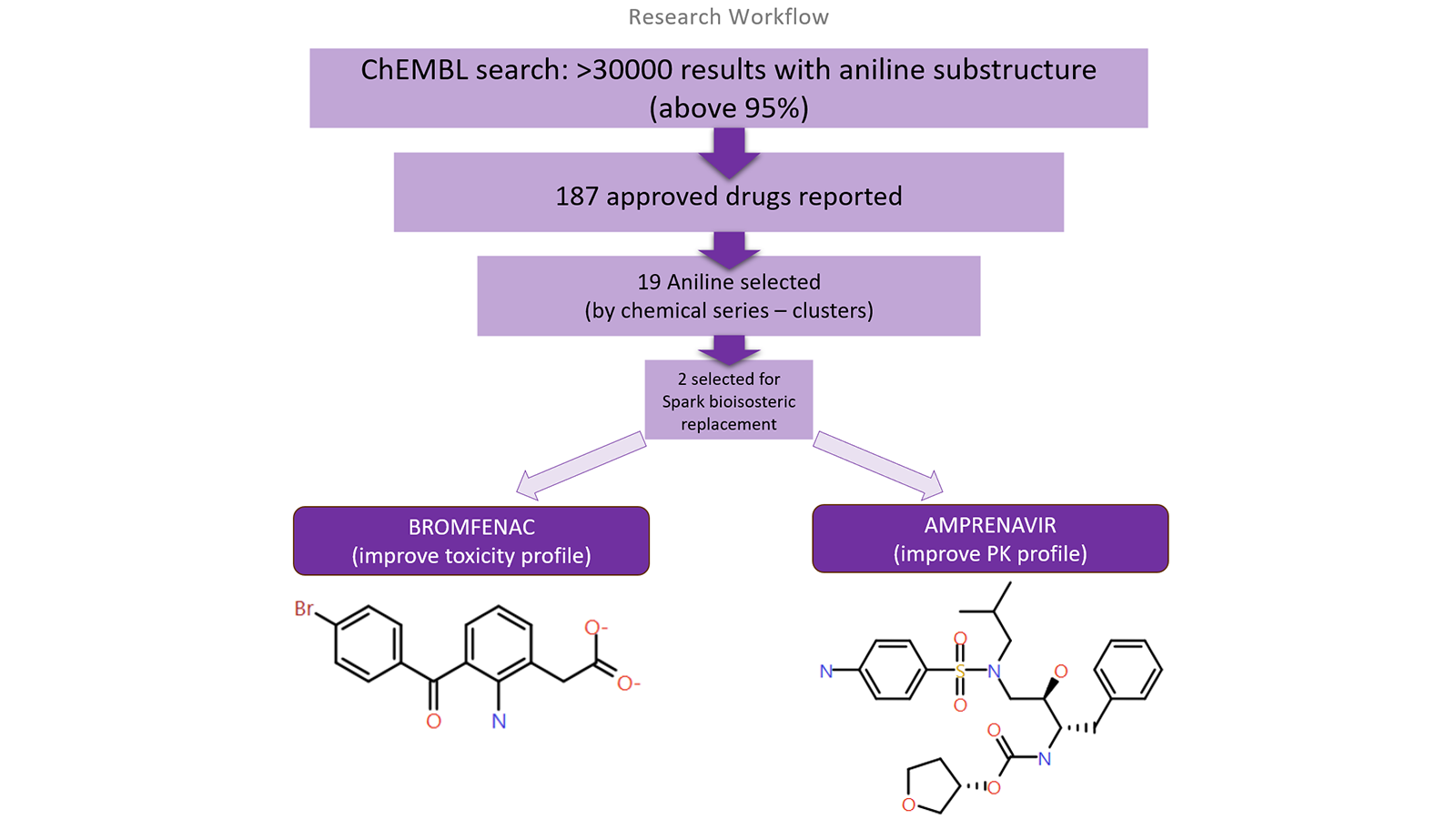
Figure 1. A research workflow to explore the compounds containing an aniline chemical group.
Using Spark, small bioisosteric replacements of the anilines were identified. The fragment databases searched were the ChEMBL2 and Enamine (Commercial Very Common and Common) fragments databases which provide more than 200,000 alternatives to search. Spark field and shape search were performed and the results from this search identified a range of bioisosteres such as benzimidazoles, substituted phenols, amides, pyrroles, pyridines fragments amongst others. A stepwise workflow is explained.
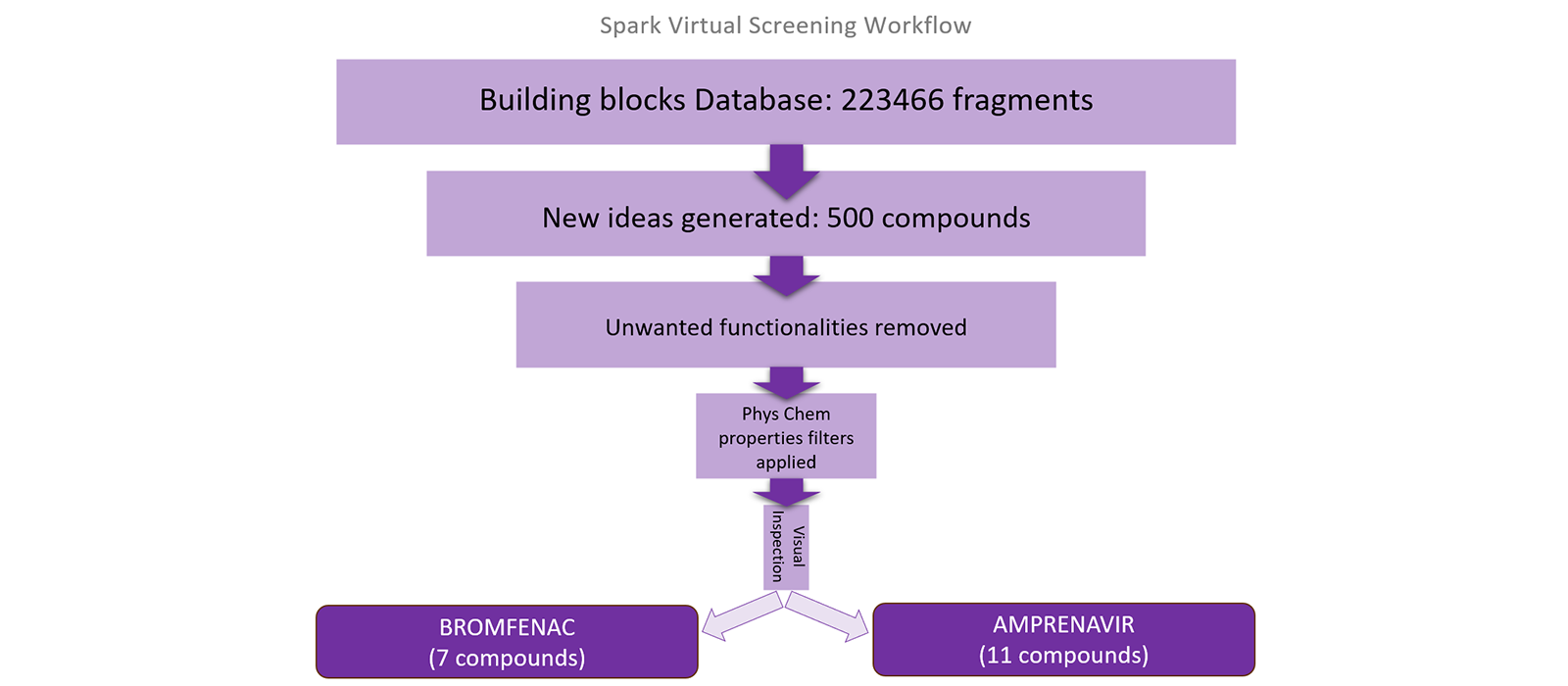
Figure 2. A Spark Virtual Screening workflow to replace an aniline chemical group.
Fine-tuning pharmacological properties
Substituting anilines with different chemical groups offers a powerful strategy for optimizing drug properties. This approach allows researchers to fine-tune various pharmacological aspects of the drug. For instance, by selecting specific chemical moieties to replace anilines, it becomes possible to enhance drug bioavailability, solubility and receptor selectivity.
AMPRENAVIR: Aniline replacement to improve PK profile
Amprenavir is a protease inhibitor used to treat HIV infection. It was approved by the FDA in 1999, with twice daily dosing. Several crystal structures have been deposited in the Protein Data Bank3 with Amprenavir co-crystalised. In 7N6X protein the ligand is bound to the protein by making several interactions in the active site of HIV Protease.4 Notably, the aniline in this case is able to interact with Asp30, therefore any replacement in this position aimed at improving the PK profile of this compound needs to be able to maintain or improve the interactions with Asp or surrounding residues.
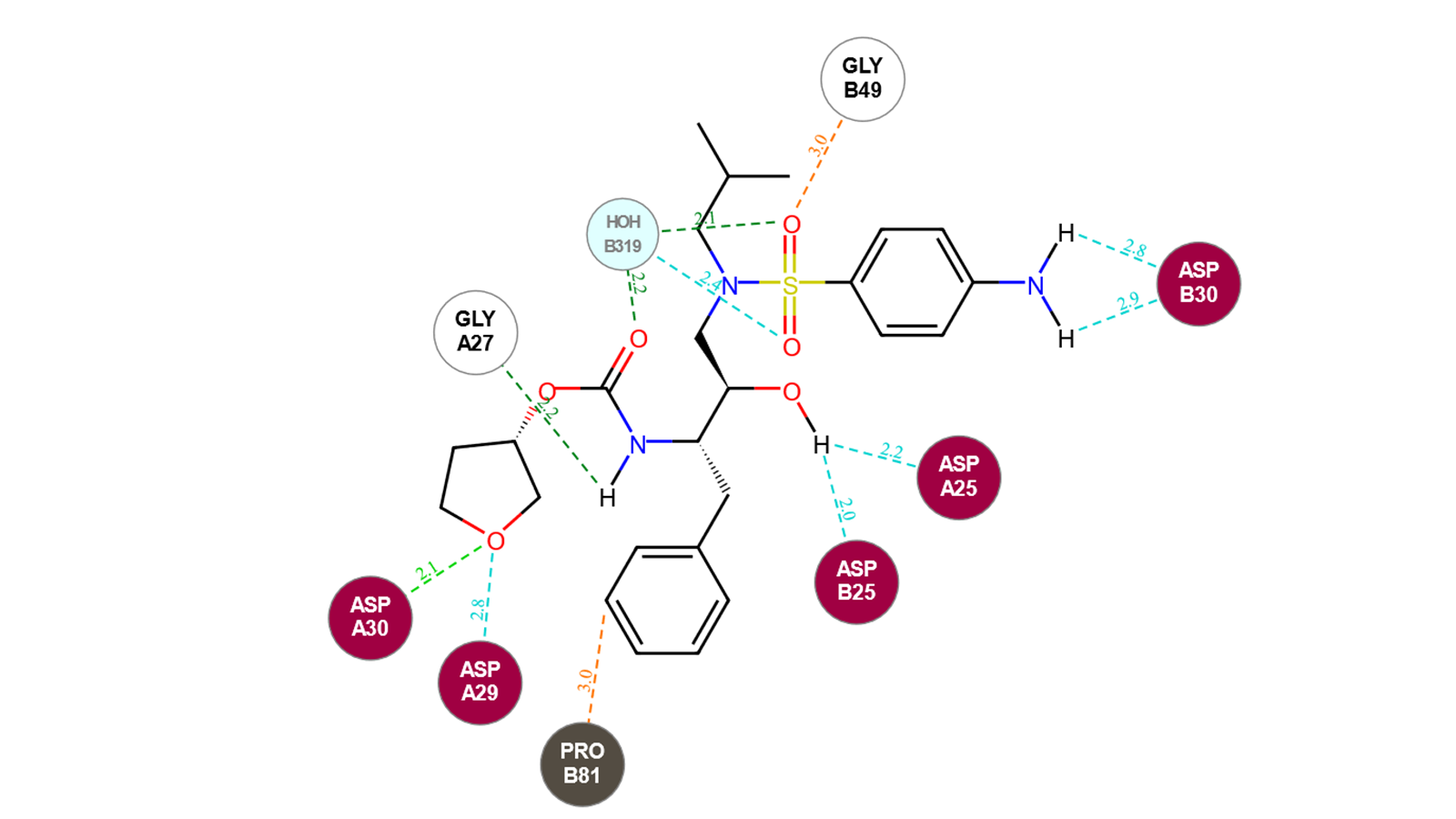
Figure 3. 2D interaction map of Amprenavir with HIV Protease active site (PDB: 7N6X).
Interactions: strong H-bonds (Donor/Acceptor distance within 2.6 Å, angle within the ideal range)→ light green, average H-bonds (Donor/Acceptor distance within 2.6 Å, angle within the allowed range) → dark green, weak H-bonds (Donor/Acceptor distance within 3 Å, Donor angle > 100°, Acceptor angle as for standard H-bonds + 20°) → cyan, steric clashes → orange, hydrophobic contacts.
The aniline group was selected as the fragment to be replaced (halo in Figure 4), with the attachment point atom (blue circle in Figure 4) selected to ensure that results of this experiment contain only aniline bioisosteres of the original ligand. An excluded volume with the protein 7N6X was used as an advanced structure-based option for this experiment.
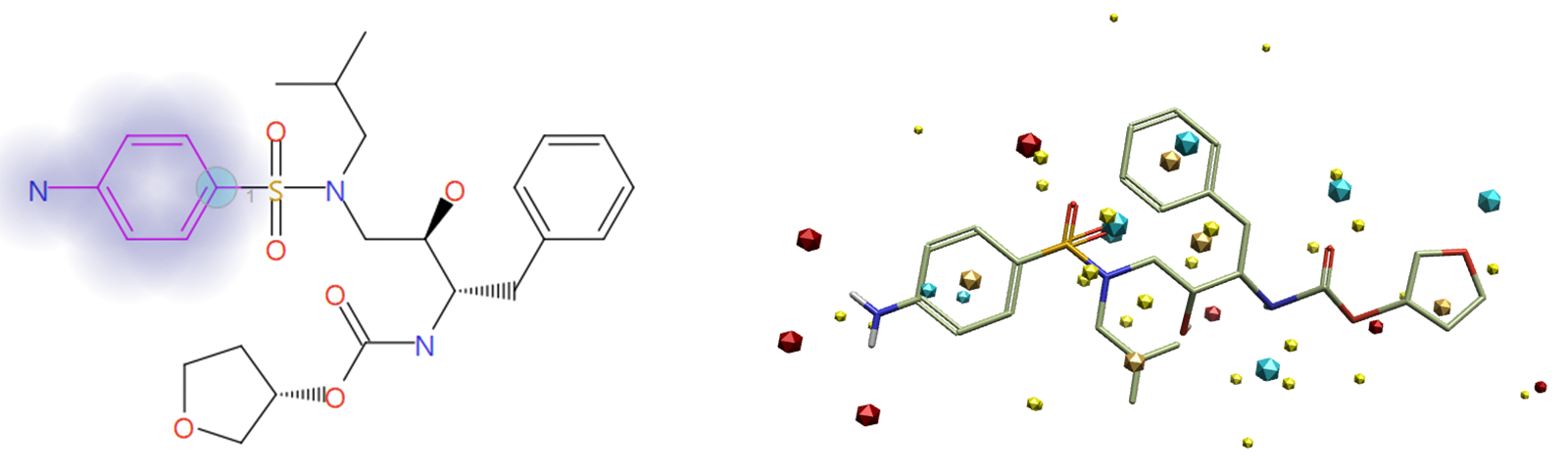
Figure 4. Aniline group selected and highlighted in halo as the fragment to be replaced, with the attachment point atom (left), and field points calculated for the ligand (right; Blue – Negative Electrostatic field, Red - Positive Electrostatic field, Orange - Hydrophobicity field, Yellow - Van der Walls field).
Among the 500 new ideas generated with Spark, 66 were selected after a strict phys-chem properties evaluation to be sure only the ones with a better PK profile were considered. Docking was performed and those compounds were ranked by LF ∆G score.5
The top-scoring 11 compounds identified are reported in Table 1. Considering that the purpose of the experiment was to find compounds with better PK profiles, compounds 3, 7 and 8 were selected as the best results from this Spark analysis. Those compounds are also able to engage with additional interactions in the active site: Compound 3 bears a primary amide that interacts with both Asp29 and Asp30 backbones with H-bond interactions, Compound 7 bears a pyrrole and establishes interaction with crystal water HOH322 and Compound 8 Isothiazole interacts with the carbonyl of Asp30 backbone.
Table 1. Selected results of Amprenavir bioistosteric replacement.
The obtained new ideas are reported, and the replacement moiety highlighted (column 4).
Properties are color coded for comparison with Amprenavir (green - better value, yellow - same value and purple - worse value). Images generated using Flare™.6
Compound |
2D |
3D |
Replacement moiety |
MW |
sLogP7 |
Rof5 (Rule of 5 violations) |
Amprenavir |
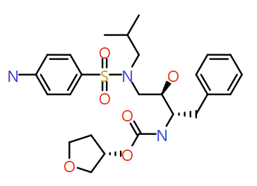 |
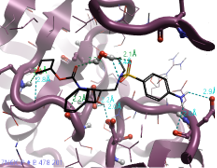 |
/ |
505.6 |
2.8 |
1 |
1 |
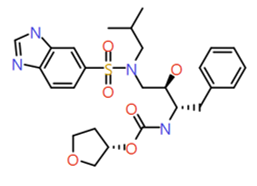 |
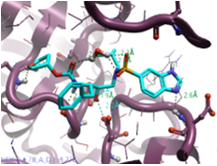 |
Benzimidazole |
530.6 |
3.1 |
1 |
2 |
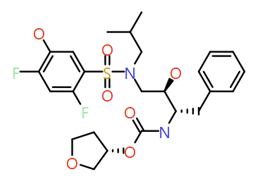 |
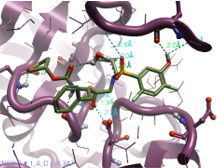 |
2,4-Difluoro phenol |
542.6 |
3.7 |
1 |
3 |
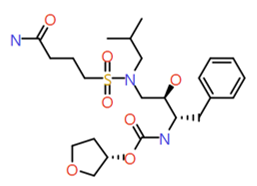 |
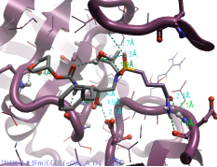 |
Butylamide |
499.6 |
1.4 |
0 |
4 |
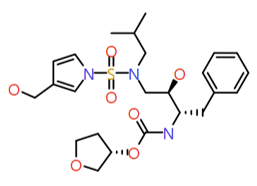 |
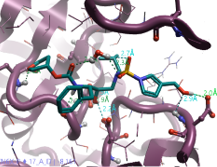 |
Pyrrol 3-yl-methanol |
509.6 |
2.3 |
1 |
5 |
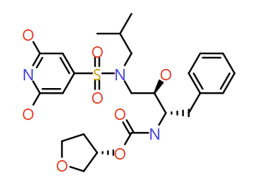 |
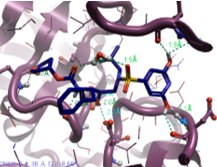 |
2,6-Dihydroxy pyridine |
523.6 |
2 |
2 |
6 |
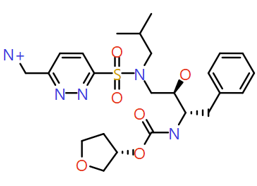 |
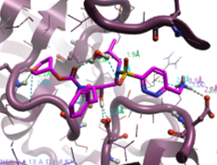 |
Pyridazin‐3‐yl-methanamine |
522.6 |
1.4 |
2 |
7 |
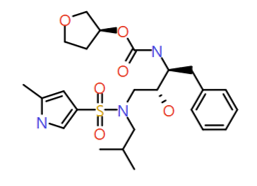 |
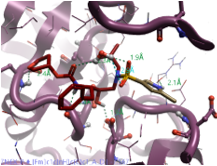 |
2-Methyl pyrrole |
493.6 |
2.8 |
0 |
8 |
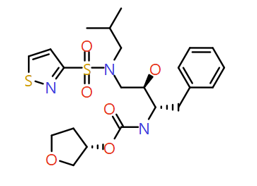 |
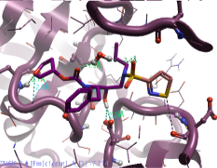 |
Isothiazole |
497.6 |
2.7 |
0 |
9 |
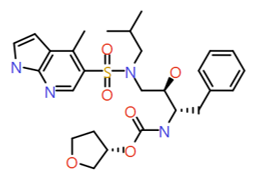 |
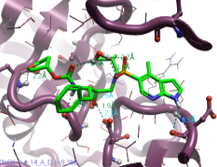 |
4-Methyl 7-azaindole |
544.6 |
3.4 |
1 |
10 |
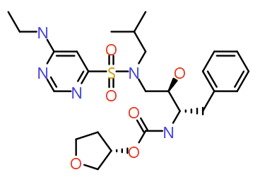 |
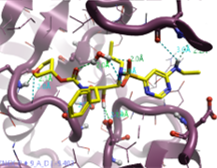 |
N‐ethylpyrimidin‐4‐amine |
535.7 |
2.4 |
2 |
11 |
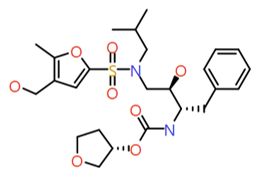 |
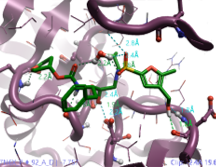 |
(2‐Methylfuran‐3‐yl)methanol |
524.6 |
2.4 |
1 |
Mitigating Toxicity and Off-Target Effects
By carefully designing and selecting alternative chemical groups, Cresset Discovery’s expert modelers can mitigate the adverse effects that anilines might cause. Additionally, chemical modifications can help improve the safety profile of drugs by reducing off-target effects. Anilines, when left unaltered, might interact with off-targets, leading to unexpected side effects. By replacing anilines with more specific and target-oriented designed chemical structures, the risk of off-target interactions can be substantially reduced, increasing the overall safety of the drug.
BROMFENAC: Aniline replacement to overcome toxicity issues
Bromfenac is a small molecule that has been an approved by the FDA as a non-steroid anti-inflammatory drug (NSAID). Its main activity is blockage of synthesis of prostaglandins through inhibition of COX-2. In the last two decades, the drug has been withdrawn from the market in some countries due to its toxicity, in particular for observed cases of severe hepatitis and liver failure (some requiring transplantation).8,9
It has been hypothesized that the toxic effects of Bromfenac were associated with metabolism. When aniline containing NSAIDs are metabolized by the liver, they can be converted into reactive metabolites. These reactive metabolites may bind to cellular proteins and other molecules in the liver, particularly cytochrome P450 enzymes, potentially causing cellular damage. These reactive metabolites can, in some cases, interfere with normal cellular processes, leading to liver injury.
The aniline group was selected as the fragment to be replaced (halo in Figure 5), with the attachment atoms (blue circles in Figure 5) selected to ensure that results of this experiment contain only aniline bioisosteres of the original ligand.
Figure 5. Aniline group selected and highlighted in halo as the fragment to be replaced, with the attachment point atom (left), and field points calculated for the ligand (right; Blue – Negative Electrostatic field, Red - Positive Electrostatic field, Orange - Hydrophobicity field, Yellow - Van der Walls field).
Among the 500 ideas generated, only 27 were drug-like compounds and, among those, the best 7 results are shown in Table 2, where the chemical diversity of the possible replacement is demonstrated.
Table 2. Selected results of Bromfenac bioistosteric replacement. The obtained new ideas are reported, and the Replacement moiety highlighted. Images generated using Flare™.
Compound |
2D |
Replacement moiety |
Bromfenac |
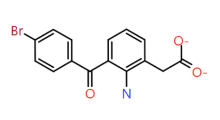 |
/ |
1 |
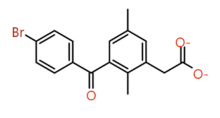 |
Di-methyl phenyl |
2 |
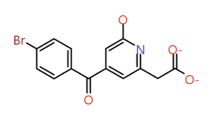 |
2-Hydroxypyridine |
3 |
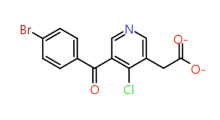 |
Para-chloro pyridine |
4 |
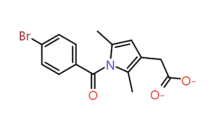 |
2,5-Dimethyl pyrrole |
5 |
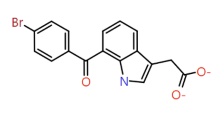 |
Indole |
6 |
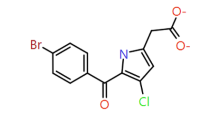 |
3-Chloro pyrrole |
7 |
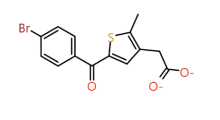 |
2-Methylthiophene |
Conclusion
Replacing anilines with other chemical groups in drug-like compounds can be a strategic decision in medicinal chemistry for several reasons. Anilines, while versatile, can exhibit undesirable properties such as metabolic instability or toxicity. By substituting anilines with different functional groups, medicinal chemists can fine-tune the compound's pharmacological properties, enhancing factors like bioavailability, solubility, or selectivity for the target receptor. These substitutions also allow for the mitigation of potential toxicities or off-target effects associated with anilines.
By addressing issues such as metabolic instability, toxicity, and off-target effects through chemical modifications, Cresset Discovery’s expert team can pave the way for safer and more effective drug therapies. It is through such innovative tools, like Spark and Flare, used in combination by our expert modelers, that the pharmaceutical industry continues to make significant strides towards better drugs.
References
- Spark™, Cresset®, Litlington, Cambridgeshire, UK; https://www.cresset-group.com/spark/; Cheeseright T., Mackey M., Rose S., Vinter, A.; Molecular Field Extrema as Descriptors of Biological Activity: Definition and Validation. J. Chem. Inf. Model. 2006, 46 (2), 665-676
- https://www.ebi.ac.uk/chembl/
- Protein Data Bank, https://www.rcsb.org/
- Revertant mutation V48G alters conformational dynamics of highly drug resistant HIV protease PRS17. Burnaman, S.H., Kneller, D.W., Wang, Y.F., Kovalevsky, A., Weber, I.T. (2021) J Mol Graph Model 108: 108005-108005
- Docking score optimized to provide an accurate estimate of protein-ligand binding energy, on the assumption that the pose is correct. Stroganov O.V. et al., Lead Finder: an approach to improve accuracy of protein-ligand docking, binding energy estimation, and virtual screening, J. Chem. Inf. Model. 2008, 48(12), 2371-2385
- Flare™, Cresset®, Litlington, Cambridgeshire, UK; https://www.cresset-group.com/flare/; Cheeseright T., Mackey M., Rose S., Vinter, A.; Molecular Field Extrema as Descriptors of Biological Activity: Definition and Validation J. Chem. Inf. Model. 2006, 46 (2), 665-676
- Wildman-Crippen calculated logP. This value is derived by applying the rules of Wildman and Crippen and represents a good estimate of the actual logP of the molecule. S. A. Wildman, G. M. Crippen, Prediction of Physicochemical Properties by Atomic Contributions, J. Chem. Inf. Comput. Sci., 1999, 39, 868-873
- Wan Po, AL. (2007). Postmarketing Surveillance. In Comprehensive Medicinal Chemistry II (pp. 755-777). Elsevier
- Code of Federal Regulations 216.24: Drug products withdrawn or removed from the market for reasons of safety or effectiveness